Objective: The aim of this randomized, sham-controlled study was to investigate the therapeutic effects of underlying neurobiological changes after 2-week repetitive transcranial magnetic stimulation (rTMS) treatment using functional connectivity magnetic resonance imaging in patients with major depression.
Methods: Twenty-four patients with major depressive disorder diagnosed with DSM-IV-TR criteria were randomly assigned to the active rTMS (n = 13) or sham (n = 11) groups from January 2009 to June 2011. rTMS was given for 2 weeks at 110% of the motor threshold for 10 minutes at 10 Hz over the left dorsolateral prefrontal cortex (DLPFC). Resting state functional connectivity was evaluated before and after rTMS. The 17-item Hamilton Depression Rating Scale (HDRS) was administered, and neurocognitive tasks were performed. We examined between-group differences in functional connectivity changes from the bilateral DLPFC.
Results: Participants in the active rTMS group showed significant clinical improvement in HDRS scores compared to those in the sham group (P < .001). After 2-week rTMS, there were significant differences in changes in DLPFC-left caudate connectivity (corrected P < .05): the active group showed a greater reduction of connectivity strength between the DLPFC and left caudate compared to the sham group. Reduced levels of DLPFC-left caudate connectivity predicted improvement in depressive symptoms (r = 0.58, P = .001). Additionally, a positive correlation between residual depressive symptoms and connectivity strength after 2-week rTMS was found (r = 0.46, P = .023).
Conclusions: High-frequency rTMS over the left DLPFC showed therapeutic effects in patients with major depression. The therapeutic effect of rTMS is related to the modulation of functional connectivity in the frontostriatal network.
Trial Registration: ClinicalTrials.gov identifier: NCT01325831‘ ‹
Objective: The aim of this randomized, sham-controlled study was to investigate the therapeutic effects of underlying neurobiological changes after 2-week repetitive transcranial magnetic stimulation (rTMS) treatment using functional connectivity magnetic resonance imaging in patients with major depression.
Methods: Twenty-four patients with major depressive disorder diagnosed with DSM-IV-TR criteria were randomly assigned to the active rTMS (n = 13) or sham (n = 11) groups from January 2009 to June 2011. rTMS was given for 2 weeks at 110% of the motor threshold for 10 minutes at 10 Hz over the left dorsolateral prefrontal cortex (DLPFC). Resting state functional connectivity was evaluated before and after rTMS. The 17-item Hamilton Depression Rating Scale (HDRS) was administered, and neurocognitive tasks were performed. We examined between-group differences in functional connectivity changes from the bilateral DLPFC.
Results: Participants in the active rTMS group showed significant clinical improvement in HDRS scores compared to those in the sham group (P < .001). After 2-week rTMS, there were significant differences in changes in DLPFC-left caudate connectivity (corrected P < .05): the active group showed a greater reduction of connectivity strength between the DLPFC and left caudate compared to the sham group. Reduced levels of DLPFC-left caudate connectivity predicted improvement in depressive symptoms (r = 0.58, P = .001). Additionally, a positive correlation between residual depressive symptoms and connectivity strength after 2-week rTMS was found (r = 0.46, P = .023).
Conclusions: High-frequency rTMS over the left DLPFC showed therapeutic effects in patients with major depression. The therapeutic effect of rTMS is related to the modulation of functional connectivity in the frontostriatal network.
Trial Registration: ClinicalTrials.gov identifier: NCT01325831
J Clin Psychiatry 2016;77(9):e1137-e1143
dx.doi.org/10.4088/JCP.15m10110
© Copyright 2016 Physicians Postgraduate Press, Inc.
aInstitute of Behavioral Science in Medicine & Department of Psychiatry, Yonsei University College of Medicine, Seoul, South Korea
bNeuroscience Research Institute, Seoul National University Medical Research Center, Seoul, South Korea
cDepartment of Adolescent Psychiatry, Seoul National Hospital, Seoul, South Korea
dDepartment of Neurosurgery, St Peter’s Hospital, Seoul, South Korea
eDepartment of Biomedical Engineering, Hanyang University, Seoul, South Korea
‡Drs Kang and H. Lee contributed equally in the conception of the study and writing of the manuscript.
*Corresponding author: Eun Lee, MD, PhD, Department of Psychiatry, Yonsei University College of Medicine, Yonsei-ro 50, Seodaemun-gu, Seoul 03722, South Korea ([email protected]).
Transcranial magnetic stimulation (TMS) is a noninvasive technique to stimulate the human brain.1 It involves the delivery of magnetic pulses through the skull to focused regions of the cortex and induces a focal electrical current in the underlying tissue and localized neuronal depolarization. The induced TMS field is estimated to be 2 cm below the scalp, reflecting the local stimulation effects of the underlying cortex near the gray-white junction2 and the remote effects of related neurocircuitry by propagating transynaptically to functionally interconnected brain areas.3,4 Through these effects, repetitive TMS (rTMS) is able to alter brain neurophysiology5 and thus may be a promising modality for modulating neurobiological abnormalities in neuropsychiatric disorders, particularly in major depression.
Data from controlled trials and meta-analyses suggest that rTMS is an effective treatment tool for major depression.6,7 Notably, rTMS can be a propitious treatment option for patients with treatment-resistant depression who do not benefit from pharmacologic approaches.8 The dorsolateral prefrontal cortex (DLPFC) has been targeted in most rTMS trials for depression because it has been implicated in the pathophysiology of major depression.9 In particular, the left DLPFC, which has lower metabolic activity,6 has been considered a valid rTMS target site. Clinical evidence has shown substantial antidepressant effects from high-frequency stimulation over the left DLPFC in major depression.10,11 However, the neurobiological mechanisms underlying the antidepressant effect of rTMS over the DLPFC remain unclear.
Previous functional neuroimaging studies demonstrated that an impaired frontostriatal network is an essential deficit in treatment-resistant depression.12,13 A recent study showed that impaired frontostriatal connectivity predicted a better outcome after rTMS treatment.14 Additionally, an impaired frontostriatal network is also observed in drug-naive early depression patients.15 Other studies that evaluated the therapeutic mechanism of rTMS focused on the DLPFC and subgenual anterior cingulate cortex.16-19 However, as suggested by Mayberg et al,20 the therapeutic effects of antidepressant treatment have some placebo effects regardless of the mode of treatment. Most previous studies examining the mechanisms of rTMS on depression did not use a sham-controlled design.14,17-19 Therefore, we conducted a randomized, rater-blind, sham-controlled study to evaluate the specific treatment effects of rTMS.16-19,21
Moreover, there is little evidence regarding the therapeutic effects of rTMS with a seed region of DLPFC. The antidepressant effects of rTMS may be associated with a cascade of neurobiological changes in brain regions that are linked with the stimulated area. Based on the anatomic and functional brain connectivity, the left DLPFC receives input from specific sensory cortices and has dense interconnections with the subcortical areas involved in emotional and cognitive modulation, such as the limbic area and the striatum.22,23 Thus, the evaluation of changes in their connectivity from the stimulated area would provide clinical evidence for rTMS of the DLPFC in treatment-resistant depression.
The purpose of the present randomized, sham-controlled study was to investigate the possible therapeutic effects of and the underlying neurobiological changes with a 2-week high-frequency rTMS treatment on the left DLPFC in patients with treatment-resistant major depression. We hypothesized that changes in functional connectivity of the frontostriatal circuitry are critical for the pathophysiology and therapeutic effects of rTMS intervention in major depression. To address this, we performed whole-brain voxel-wise functional connectivity analyses to generate functional connectivity maps of the DLPFC for the resting-state data.
METHODS
Study Design
Participants with major depressive disorder (MDD) were randomly assigned with stratification to either the active or the sham group based on age, gender, and severity of depression symptoms. The participants and raters (K.R.K. and K.J.) were blind to the expected effects of each condition. However, the clinicians (J.M.K. and H.J.Y.) administering rTMS were aware of the treatment groups. Considering the potential risk of interaction between subjects and the nonblinded treater, limited interaction between them was requested during rTMS sessions. Power computations show that this study requires a sample size of 10 in each group, assuming 80% power with a 2-sided significance level of α = .05 if the effect size is supposed to be 1.4 from a previous study.24 The study was registered at ClinicalTrials.gov (NCT01325831).
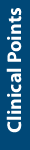
- Repetitive transcranial magnetic stimulation (rTMS), a noninvasive brain stimulation technique, is a promising modality for patients with major depression, but the neurobiological mechanisms underlying the antidepressant effect of rTMS remain unclear.
- High-frequency rTMS over the left dorsolateral prefrontal cortex is an effective treatment modality for patients with major depression, and the therapeutic effects of rTMS may be mediated by recovering the impaired frontostriatal network functional connectivity.
Participants
Via postings in the hospital and local newspaper advertisements, 28 right-handed outpatients were recruited from the depression clinic at Severance Hospital in Seoul, South Korea from January 2009 to June 2011. Trained psychiatrists (K.R.K. and K.J.) confirmed the DSM-IV-TR25 diagnostic criteria for MDD in patients presenting with a current major depressive episode using the Mini-International Neuropsychiatric Interview.26 Participants ranged in age from 20 to 75 years and had failed to achieve adequate improvement (less than 50% improvement evaluated by the 17-item Hamilton Depression Rating Scale [HDRS]27) after at least 8 weeks of treatment with at least 1 serotonin reuptake inhibitor. Participants were excluded if they presented with any current psychiatric disorder other than MDD, including anxiety disorder and substance use disorder (except nicotine). We also excluded any participants having a current or past history of psychotic disorder, seizure, mental retardation, high risk of suicide, cognitive impairment (score < 24 on the Mini-Mental State Examination),28 or contraindications for general functional magnetic resonance imaging (fMRI) experiments. While all participants were taking antidepressants for at least 8 weeks before the MRI scan, the pharmacologic regimen did not change during the study period. To confirm the preservation of blinding, we asked 2 questions after the 10 sessions of rTMS: “Do you know the treatment condition you’ ve received?” and “Which condition do you think you received?” The institutional review board of Severance Hospital approved the study, and written informed consent was obtained from all participants before participation.
Stimulation Parameters
A Magstim rapid magnetic stimulator with a 70-mm figure-of-eight coil (Whitland, UK) was used for the treatment. Patients received 10 daily rTMS sessions for 2 weeks. Before the first session, the resting motor threshold (RMT) was determined over the primary motor cortex by determining the minimal intensity required to elicit at least 5 motor-evoked potentials of 50 μV out of 10 stimulations of the abductor pollicis brevis. For the active group, rTMS over the left DLPFC was administered at the intensity of 110% of the RMT, a frequency of 10 Hz for 5 seconds per train, and 20 trains with an intertrain interval of 25 seconds for 10 minutes (1,000 stimuli/d). The rTMS was conducted at a point 5 cm anterior to the point at which the RMT was determined. For the sham group, stimulation was applied with D70-mm α coil with a double, figure-of-eight shape produced by Magstim Co (Whitland, UK), which makes no difference in terms of scalp sensations and sound generated by the active and sham rTMS coil.29
Assessment of Clinical Symptoms and Neurocognitive Function
Clinical and neurocognitive evaluations were performed 4 days before the first rTMS session and 4 days after the final rTMS session. Depressive symptoms were evaluated using the HDRS. “Response” to rTMS was defined by at least 50% improvement from the baseline HDRS score. “Remission” was defined as a final HDRS score below 9.
Neurocognitive function, particularly frontal lobe function, was assessed using the Rey Auditory Verbal Learning Test,30 the Stroop test,31,32 and the backward digit span and digit symbol tests from the Wechsler Adult Intelligence Scale, Third Edition.33
Functional Connectivity MRI Procedures and Data Analyses
Full methodological details of the functional connectivity MRI (fcMRI) data are described in eAppendix 1. Preprocessing and statistical analyses of the fcMRI data were performed using the Analysis of Functional NeuroImages software.34 After preprocessing, individual functional connectivity maps were produced by correlation analysis between the average blood oxygen level-dependent time course extracted from the seed region of interest (ROI) of bilateral DLPFC and the time courses from all other brain voxels. The seed region is shown in Figure 1. Then, we obtained individual contrast maps that demonstrated changes in functional connectivity strength before and after rTMS sessions. To examine group differences, we performed 2-sample t tests on contrast maps between the active and the sham groups. Statistically defined clusters of activation were identified at corrected P < .05. In the post hoc analysis, we examined hemispheric effect of the seed ROI on results of the group difference of the functional connectivity. In addition, Spearman correlation analyses were conducted to explore how functional connectivity changes on the survived clusters in group analyses were related to the depression severity or neurocognitive function. To verify the accuracy and robustness of the results, jackknife procedures were used.
RESULTS
Demographic and Clinical Characteristics
After the screening interview, 2 participants did not reach the criteria of a current major depressive episode. Another 2 participants had comorbid anxiety disorder and alcohol use disorder. Therefore, a total of 24 eligible patients with treatment-resistant major depression were randomly assigned to the active rTMS (n = 13) or sham (n = 11) groups. One participant withdrew the informed consent after 1 stimulation session due to headache. Another 2 participants failed to complete the 10 sessions of rTMS due to malfunction of the rTMS machine. Thus, the final analyses included 12 patients in the active group and 9 in the sham group. Among the 21 subjects, most of them (n = 16) were on selective serotonin reuptake inhibitor (SSRI) monotherapy (7 escitalopram, 6 fluoxetine, 1 sertraline, and 2 paroxetine), and 5 patients were taking an SSRI in combination with another antidepressant: escitalopram + bupropion (n = 4) and escitalopram + venlafaxine (n = 1). There were no significant differences at baseline in the demographic and clinical variables between participants in the active and sham groups (Table 1). For the response to the blindness awareness questions, no difference was found between the 2 groups.
Regarding tolerability and safety, there were no seizures observed in any participant. With the exception of the 1 participant who dropped out due to transient headache, no serious side effects were reported during the session. The withdrawal rate due to all reasons during the study was 4.5%.
Clinical Effect of rTMS Treatment
For the 2-week rTMS treatment, repeated-measures analyses of variance on all participants showed a significant time effect on depression severity (F = 96.2, P < .001) within subjects. A significant group-by-time interaction was observed for depression severity (F = 24.1, P < .001). Specifically, the active group showed greater improvement in depressive symptoms compared to the sham group. Among the active group, 9 of 12 participants showed responses to rTMS, whereas no participants responded to the sham stimulation (P < .001). The remission rate was 25% (n = 3) in the active group and 0% in the sham group; however, the group difference did not reach statistical significance (P = .229). Performance on all neurocognitive tasks did not show group-by-time interactions (Table 2).
Effect of rTMS on Functional Connectivity
Changes in the functional connectivity from the DLPFC were significantly different in the left caudate (peak at Montreal Neurological Institute and Hospital [MNI] coordinates: −14, 14, 18; cluster size: 296 mm3) between the active and the sham groups (Figure 1). The post hoc analysis revealed no significant interaction effect by seed hemisphere and groups in the left caudate (F = 3.056, P = .097), which reflects no hemispheric effect of the seed ROI to results of the group difference. The active group showed a significantly greater reduction in connectivity strength between the DLPFC and left caudate compared to the sham group (see Figure 1). The significant difference was still robust in the 2-sample t test using a jackknife approach (see Figure 1).
Spearman analyses showed that reduced functional connectivity strength between the left caudate and the DLPFC (pre-TMS minus post-TMS) predicted improvement in depression severity (jackknife estimated r = 0.58, P = .001). Moreover, the residual depressive symptoms after 2-week rTMS were significantly positively correlated with the connectivity strength between the left caudate and the DLPFC (jackknife estimated r = 0.46, P = .023). Changes in functional connectivity strength within the groups were not related to the changes in neurocognitive function.
DISCUSSION
This randomized, sham-controlled study examined the therapeutic effects and underlying neurobiological effects of rTMS in patients with treatment-resistant major depression using fcMRI. The present study demonstrated the therapeutic mechanism of high frequency rTMS over the left DLPFC, which is mediated via modulation of the frontostriatal network.
In our study, the active group exhibited greater improvement in depressive symptoms compared to the sham group. The fcMRI results showed that changes between the DLPFC and left caudate revealed a significant difference between active and sham groups, and the connectivity change significantly correlated with improved HDRS scores during the 2-week rTMS treatment. These findings suggest that the therapeutic effect of rTMS over the left DLPFC in major depression is related to the recovery of impaired connectivity between the DLPFC and left caudate. Moreover, residual depressive symptoms after the 2-week rTMS correlated with connectivity strength between the left caudate and the bilateral DLPFC. This result provides clinical implications for the altered functional connectivity between the DLPFC and the caudate as a neural correlate of treatment-resistant major depression. However, this result could be narrowly interpreted as one of the various connectivity changes that occur while the therapeutic process is ongoing. Because this study targeted patients with inadequate response to medication, our results may simply reflect the therapeutic mechanisms of the subpopulation with MDD that is unresponsive to pharmacologic treatment. Additional studies may be required to verify whether changes in frontostriatal connectivity are the general therapeutic mechanism of rTMS for depression.
Our findings have implications for clinical importance of the caudate in patients with major depression. The caudate is known to be involved in reward-related learning and motivation.35 Neuroanatomically, the caudate is a major receptive part of the striatum with neural innervation from various cortical areas, including the prefrontal cortex and thalamus.36 Converging lines of evidence from clinical studies also support the role of the caudate in depression. A study of the resting-state default mode network in drug-naive individuals showed that decreased connectivity between the caudate and the precuneus/posterior cingulate cortex was a neural correlate of major depression.15 Recently, 2 studies demonstrated that an increased functional connectivity between the DLPFC and caudate correlated with depression severity.37,38 One study was conducted in young, medication-free, depressed patients.38 Moreover, the association between frontostriatal atrophy and poor outcome of treatment was found,39 and reduced gray matter volumes in the caudate were reported in the patients with treatment-resistant depression,13 which suggested the involvement of the caudate in treatment resistance and chronicity of major depression. A recent prospective rTMS trial showed that abnormal frontostriatal connectivity predicted clinical improvement after rTMS treatment over the dorsomedial PFC.14 Collectively, these findings indicate that frontostriatal circuit abnormalities may be the core deficits involved in the determination of treatment response as well as a neural correlate of major depression.
Our significant finding was confined only to the left caudate. The left-lateralized caudate effect may be derived from the administration of left-lateralized prefrontal rTMS treatment. Substantial evidence implicates left hemisphere involvement in pathophysiology and symptomatic correlation in major depression. A proton magnetic resonance spectroscopy study showed that left-lateralized abnormalities in the caudate were involved in adolescents with major depression.40 A structural MRI study in adults with major depression found that baseline depressive symptoms are inversely correlated with left caudate volume.41 Moreover, change in regional cerebral blood flow of the left caudate and left prefrontal cortex correlated with the emergence of depressive symptoms after interruption of SSRI treatment.42 Taken together, these findings suggest the potential role of the left-lateralized caudate and prefrontal cortex in the pathophysiology and treatment response of depression.
On the other hand, no difference in connectivity to the subgenual cingulate cortex was found between the 2 groups. Unlike our findings, some studies reported that the subgenual anterior cingulate cortex is a crucial neural substrate for therapeutic response to rTMS in depression.16,17,19 Connectivity changes in the subgenual cingulate cortex may be related to nonspecific beneficial effects in depression, considering that subgenual anterior cingulate changes reflect a common pathway for the treatment response in depression.17,20,43 In addition, because the anterior cingulate cortex is reported to be involved in the placebo effects for depression,20 the placebo effects in the sham group may negate the potential changes in the subgenual cingulate cortex in our sham-controlled study.
It should be noted that the clinical improvement in our depressed patients was independent of cognitive performance changes. In addition, changes in neurocognitive function were not related to the changes in the frontostriatal connectivity strength. Based on a recent review,44 it is still difficult to draw clear conclusion about neurocognitive effects and the underlying mechanism of rTMS, although there have been many studies supporting the beneficial effect on cognitive functions. Several reports revealed that cognitive improvement during the rTMS treatment period could not be explained by mood improvement.45,46 The potential effects of rTMS on neurocognitive function may be mediated through different neural circuits from those predicting improvement of depressive symptoms.44,46
Several limitations of our study should be mentioned. First, the sample size of each group was relatively small. The small sample size may have led to false-negative errors and limited our statistical power to detect subtle connectivity abnormalities, including anterior cingulate, thalamus, and other limbic areas, as well as correlations with clinical or cognitive symptoms. Second, participants enrolled in our study had a wide range of ages. However, there were no significant differences between the active and the sham groups. Age-related declines in neural plasticity may reduce the therapeutic effects of rTMS treatment. Third, the standard 5 cm target may be less effective than the individualized positioning methods or TMS positioning based on anatomic coordinates.17,47 Advanced techniques for optimal TMS targeting should help enhance the targeting accuracy and reduce intersubject variability in placement. Fourth, in the present design with sham stimulation at the same location as a control, we cannot conclude whether the finding of the frontostriatal change is specific to rTMS over the left DLPFC or whether they would have been also related to rTMS to different cortical targets. Finally, the present findings using 2-week rTMS protocol should be interpreted with caution and their application restricted to the acute effects of prefrontal rTMS. In future research, a longer treatment period would be required for greater clinical benefits or long-lasting effects.
In summary, high-frequency rTMS treatment over the left DLPFC had therapeutic effects in patients with treatment-resistant major depression. The neurobiological mechanism of the therapeutic effects was associated with changes in the resting functional connectivity between the DLPFC and the left caudate. This finding suggests that the antidepressant effects of rTMS may be mediated by the modulation of the frontostriatal network. Our results have implications for understanding the efficacious clinical application of rTMS for patients with major depression as well as the underlying neural correlates involved in neurobiological changes after rTMS treatment.
Submitted: May 15, 2015; accepted October 29, 2015.
Online first: July 5, 2016.
Drug names: bupropion (Wellbutrin and others), escitalopram (Lexapro and others), fluoxetine (Prozac and others), paroxetine (Paxil, Pexeva, and others), sertraline (Zoloft and others).
Potential conflicts of interest: The authors report no financial or other relationship relevant to the subject of this article.
Funding/support: This work was supported by a faculty research grant from the Yonsei University College of Medicine (6-2013-0180).
Role of the sponsor: The funding source had no influence on the study design, data collection, analysis, interpretation of data, writing of the report, or the decision to submit the paper for publication.
Acknowledgments: We would like to thank Jae Min Kim, MD, of Sungnam Sarang Hospital (Sungnam, South Korea) and Hye Jin Yoon, MD, of Bucheon Korea Hospital (Bucheon, South Korea) for their assistance in administering rTMS. Drs Kim and Yoon have no conflicts of interest relevant to the subject of this article.
Supplementary material: See accompanying pages.
REFERENCES
1. Hallett M. Transcranial magnetic stimulation and the human brain. Nature. 2000;406(6792):147-150. PubMed doi:10.1038/35018000
2. Epstein CM, Schwartzberg DG, Davey KR, et al. Localizing the site of magnetic brain stimulation in humans. Neurology. 1990;40(4):666-670. PubMed doi:10.1212/WNL.40.4.666
3. Speer AM, Willis MW, Herscovitch P, et al. Intensity-dependent regional cerebral blood flow during 1-Hz repetitive transcranial magnetic stimulation (rTMS) in healthy volunteers studied with H215O positron emission tomography, II: effects of prefrontal cortex rTMS. Biol Psychiatry. 2003;54(8):826-832. PubMed doi:10.1016/S0006-3223(03)00324-X
4. Denslow S, Lomarev M, George MS, et al. Cortical and subcortical brain effects of transcranial magnetic stimulation (TMS)-induced movement: an interleaved TMS/functional magnetic resonance imaging study. Biol Psychiatry. 2005;57(7):752-760. PubMed doi:10.1016/j.biopsych.2004.12.017
5. Hoffman RE, Cavus I. Slow transcranial magnetic stimulation, long-term depotentiation, and brain hyperexcitability disorders. Am J Psychiatry. 2002;159(7):1093-1102. PubMed doi:10.1176/appi.ajp.159.7.1093
6. George MS, Lisanby SH, Avery D, et al. Daily left prefrontal transcranial magnetic stimulation therapy for major depressive disorder: a sham-controlled randomized trial. Arch Gen Psychiatry. 2010;67(5):507-516. PubMed doi:10.1001/archgenpsychiatry.2010.46
7. Berlim MT, Van den Eynde F, Daskalakis ZJ. High-frequency repetitive transcranial magnetic stimulation accelerates and enhances the clinical response to antidepressants in major depression: a meta-analysis of randomized, double-blind, and sham-controlled trials. J Clin Psychiatry. 2013;74(2):e122-e129. PubMed doi:10.4088/JCP.12r07996
8. George MS, Post RM. Daily left prefrontal repetitive transcranial magnetic stimulation for acute treatment of medication-resistant depression. Am J Psychiatry. 2011;168(4):356-364. PubMed doi:10.1176/appi.ajp.2010.10060864
9. Koenigs M, Grafman J. The functional neuroanatomy of depression: distinct roles for ventromedial and dorsolateral prefrontal cortex. Behav Brain Res. 2009;201(2):239-243. PubMed doi:10.1016/j.bbr.2009.03.004
10. Kozel FA, George MS. Meta-analysis of left prefrontal repetitive transcranial magnetic stimulation (rTMS) to treat depression. J Psychiatr Pract. 2002;8(5):270-275. PubMed doi:10.1097/00131746-200209000-00003
11. Anderson IM, Delvai NA, Ashim B, et al. Adjunctive fast repetitive transcranial magnetic stimulation in depression. Br J Psychiatry. 2007;190(6):533-534. PubMed doi:10.1192/bjp.bp.106.028019
12. Li CT, Chen LF, Tu PC, et al. Impaired prefronto-thalamic functional connectivity as a key feature of treatment-resistant depression: a combined MEG, PET and rTMS study. PLoS ONE. 2013;8(8):e70089. PubMed doi:10.1371/journal.pone.0070089
13. Ma C, Ding J, Li J, et al. Resting-state functional connectivity bias of middle temporal gyrus and caudate with altered gray matter volume in major depression. PLoS ONE. 2012;7(9):e45263. PubMed doi:10.1371/journal.pone.0045263
14. Salomons TV, Dunlop K, Kennedy SH, et al. Resting-state cortico-thalamic-striatal connectivity predicts response to dorsomedial prefrontal rTMS in major depressive disorder. Neuropsychopharmacology. 2014;39(2):488-498. PubMed doi:10.1038/npp.2013.222
15. Bluhm R, Williamson P, Lanius R, et al. Resting state default-mode network connectivity in early depression using a seed region-of-interest analysis: decreased connectivity with caudate nucleus. Psychiatry Clin Neurosci. 2009;63(6):754-761. PubMed doi:10.1111/j.1440-1819.2009.02030.x
16. Baeken C, Marinazzo D, Wu GR, et al. Accelerated HF-rTMS in treatment-resistant unipolar depression: Insights from subgenual anterior cingulate functional connectivity. World J Biol Psychiatry. 2014;15(4):286-297. PubMed doi:10.3109/15622975.2013.872295
17. Fox MD, Buckner RL, White MP, et al. Efficacy of transcranial magnetic stimulation targets for depression is related to intrinsic functional connectivity with the subgenual cingulate. Biol Psychiatry. 2012;72(7):595-603. PubMed doi:10.1016/j.biopsych.2012.04.028
18. Li CT, Wang SJ, Hirvonen J, et al. Antidepressant mechanism of add-on repetitive transcranial magnetic stimulation in medication-resistant depression using cerebral glucose metabolism. J Affect Disord. 2010;127(1-3):219-229. PubMed doi:10.1016/j.jad.2010.05.028
19. Liston C, Chen AC, Zebley BD, et al. Default mode network mechanisms of transcranial magnetic stimulation in depression. Biol Psychiatry. 2014;76(7):517-526. PubMed doi:10.1016/j.biopsych.2014.01.023
20. Mayberg HS, Silva JA, Brannan SK, et al. The functional neuroanatomy of the placebo effect. Am J Psychiatry. 2002;159(5):728-737. PubMed doi:10.1176/appi.ajp.159.5.728
21. Shajahan PM, Glabus MF, Steele JD, et al. Left dorso-lateral repetitive transcranial magnetic stimulation affects cortical excitability and functional connectivity, but does not impair cognition in major depression. Prog Neuropsychopharmacol Biol Psychiatry. 2002;26(5):945-954. PubMed doi:10.1016/S0278-5846(02)00210-5
22. Ferry AT, Ongür D, An X, et al. Prefrontal cortical projections to the striatum in macaque monkeys: evidence for an organization related to prefrontal networks. J Comp Neurol. 2000;425(3):447-470. PubMed doi:10.1002/1096-9861(20000925)425:3<447::AID-CNE9>3.0.CO;2-V
23. Yeterian EH, Pandya DN. Prefrontostriatal connections in relation to cortical architectonic organization in rhesus monkeys. J Comp Neurol. 1991;312(1):43-67. PubMed doi:10.1002/cne.903120105
24. George MS, Nahas Z, Molloy M, et al. A controlled trial of daily left prefrontal cortex TMS for treating depression. Biol Psychiatry. 2000;48(10):962-970. PubMed doi:10.1016/S0006-3223(00)01048-9
25. American Psychiatric Association. Diagnostic and Statistical Manual of Mental Disorders. Fourth Edition, Text Revision. Washington, DC: American Psychiatric Association; 2000.
26. Sheehan DV, Lecrubier Y, Sheehan KH, et al. The Mini-International Neuropsychiatric Interview (M.I.N.I.): the development and validation of a structured diagnostic psychiatric interview for DSM-IV and ICD-10. J Clin Psychiatry. 1998;59(suppl 20):22-33, quiz 34-57. PubMed
27. Hamilton M. A rating scale for depression. J Neurol Neurosurg Psychiatry. 1960;23(1):56-62. PubMed doi:10.1136/jnnp.23.1.56
28. Folstein MF, Folstein SE, McHugh PR. “Mini-mental state”: a practical method for grading the cognitive state of patients for the clinician. J Psychiatr Res. 1975;12(3):189-198. PubMed doi:10.1016/0022-3956(75)90026-6
29. Rossi S, Ferro M, Cincotta M, et al. A real electro-magnetic placebo (REMP) device for sham transcranial magnetic stimulation (TMS). Clin Neurophysiol. 2007;118(3):709-716. PubMed doi:10.1016/j.clinph.2006.11.005
30. Rey A. L’ examen Clinique en Psychologie. Paris, France: Presses Universitaires de France; 1964.
31. Stroop J. Studies of interference in serial verbal reactions. J Exp Psychol. 1935;18(6):643-662. doi:10.1037/h0054651
32. Golden CJ. Stroop Color and Word Test: Manual for Clinical and Experimental Uses. Chicago, IL: Stoelting; 1978.
33. Wechsler D. Wechsler Adult Intelligence Scale (WAIS-III). San Antonio, TX: The Psychological Corporation; 1997.
34. Cox RW, Hyde JS. Software tools for analysis and visualization of fMRI data. NMR Biomed. 1997;10(4-5):171-178. PubMed doi:10.1002/(SICI)1099-1492(199706/08)10:4/5<171::AID-NBM453>3.0.CO;2-L
35. Haruno M, Kuroda T, Doya K, et al. A neural correlate of reward-based behavioral learning in caudate nucleus: a functional magnetic resonance imaging study of a stochastic decision task. J Neurosci. 2004;24(7):1660-1665. PubMed doi:10.1523/JNEUROSCI.3417-03.2004
36. Draganski B, Kherif F, Klöppel S, et al. Evidence for segregated and integrative connectivity patterns in the human Basal Ganglia. J Neurosci. 2008;28(28):7143-7152. PubMed doi:10.1523/JNEUROSCI.1486-08.2008
37. Furman DJ, Hamilton JP, Gotlib IH. Frontostriatal functional connectivity in major depressive disorder. Biol Mood Anxiety Disord. 2011;1(1):11. PubMed doi:10.1186/2045-5380-1-11
38. Kerestes R, Harrison BJ, Dandash O, et al. Specific functional connectivity alterations of the dorsal striatum in young people with depression. Neuroimage Clin. 2015;7:266-272. PubMed doi:10.1016/j.nicl.2014.12.017
39. Shah PJ, Glabus MF, Goodwin GM, et al. Chronic, treatment-resistant depression and right fronto-striatal atrophy. Br J Psychiatry. 2002;180(5):434-440. PubMed doi:10.1192/bjp.180.5.434
40. Gabbay V, Hess DA, Liu S, et al. Lateralized caudate metabolic abnormalities in adolescent major depressive disorder: a proton MR spectroscopy study. Am J Psychiatry. 2007;164(12):1881-1889. PubMed doi:10.1176/appi.ajp.2007.06122032
41. Pillay SS, Renshaw PF, Bonello CM, et al. A quantitative magnetic resonance imaging study of caudate and lenticular nucleus gray matter volume in primary unipolar major depression: relationship to treatment response and clinical severity. Psychiatry Res. 1998;84(2-3):61-74. PubMed doi:10.1016/S0925-4927(98)00048-1
42. Henry ME, Kaufman MJ, Hennen J, et al. Cerebral blood volume and clinical changes on the third day of placebo substitution for SSRI treatment. Biol Psychiatry. 2003;53(1):100-105. PubMed doi:10.1016/S0006-3223(02)01441-5
43. Benedetti F, Mayberg HS, Wager TD, et al. Neurobiological mechanisms of the placebo effect. J Neurosci. 2005;25(45):10390-10402. PubMed doi:10.1523/JNEUROSCI.3458-05.2005
44. Serafini G, Pompili M, Belvederi Murri M, et al. The effects of repetitive transcranial magnetic stimulation on cognitive performance in treatment-resistant depression: a systematic review. Neuropsychobiology. 2015;71(3):125-139. PubMed doi:10.1159/000381351
45. Martis B, Alam D, Dowd SM, et al. Neurocognitive effects of repetitive transcranial magnetic stimulation in severe major depression. Clin Neurophysiol. 2003;114(6):1125-1132. PubMed doi:10.1016/S1388-2457(03)00046-4
46. Kedzior KK, Rajput V, Price G, et al. Cognitive correlates of repetitive transcranial magnetic stimulation (rTMS) in treatment-resistant depression—a pilot study. BMC Psychiatry. 2012;12(1):163. PubMed doi:10.1186/1471-244X-12-163
47. Johnson KA, Baig M, Ramsey D, et al. Prefrontal rTMS for treating depression: location and intensity results from the OPT-TMS multi-site clinical trial. Brain Stimulat. 2013;6(2):108-117. PubMed doi:10.1016/j.brs.2012.02.003
Please sign in or purchase this PDF for $40.00.
Save
Cite