Venlafaxine and O-Desmethylvenlafaxine Concentrations in Plasma and Cerebrospinal Fluid
ABSTRACT
Objective: To investigate whether drug concentrations of venlafaxine and its metabolite O-desmethylvenlafaxine in plasma can be considered as a surrogate marker of concentrations in brain/cerebrospinal fluid (CSF).
Method: For therapeutic drug monitoring purposes, plasma and CSF concentrations of venlafaxine and O-desmethylvenlafaxine were measured between November 2011 and August 2013 in 16 depressive inpatients (ICD-10 diagnoses) who were treated with daily doses of venlafaxine extended release (dose range, 75-225 mg). Daily doses were correlated with plasma and CSF levels. The correlation between venlafaxine, O-desmethylvenlafaxine, and the active moiety (AM) in plasma and CSF was calculated.
Results: Venlafaxine in plasma (P = .005) and CSF (P = .023) correlated significantly with the daily dose, while O-desmethylvenlafaxine and the active moiety (AM = venlafaxine + O-desmethylvenlafaxine) did not. The correlation between venlafaxine, O-desmethylvenlafaxine, and the AM in plasma and CSF was highly significant (P < .001). The calculated CSF/plasma ratio was 0.74 for venlafaxine, 0.88 for O-desmethylvenlafaxine, and 0.84 for the AM.
Conclusions: Venlafaxine and O-desmethylvenlafaxine were found to penetrate well into CSF in patients, which indicated good availability of the drug in the brain, although the findings on CSF concentrations do not allow calculation of concentrations at the target structure within the brain. CSF/plasma ratios for venlafaxine and its metabolite were high probably due to low plasma protein binding. The poor correlation of dose to concentrations in body fluids and the highly significant correlation of plasma to CSF concentrations indicate that plasma concentration is a much better marker of drug concentration in brain than the dose.
J Clin Psychiatry 2015;76(1):25-31
© Copyright 2014 Physicians Postgraduate Press, Inc.
Submitted: December 6, 2013; accepted February 13, 2014.
Online ahead of print: August 19, 2014 (doi:10.4088/JCP.13m08921).
Corresponding author: Michael Paulzen, MD, Department of Psychiatry, Psychotherapy, and Psychosomatics and JARA-Translational Brain Medicine, RWTH Aachen University, Pauwelsstr 30, 52074 Aachen, Germany (mpaulzen@ukaachen.de).
Drugs for the treatment of psychiatric diseases such as depression or psychosis are far from being ideal. One limitation is the poor blood-brain barrier penetration. Effective drugs must be able to penetrate the blood-brain barrier to reach their site of action within the brain. The response to psychotropic drugs is determined by the interplay of different gene products that influence pharmacokinetics and pharmacodynamics: drug-metabolizing enzymes, drug transporters, drug targets, and gene-products that are involved in post-target events.1,2 However, the exchange of compounds between blood and brain occurs not only at the blood-brain barrier, but also at the blood-cerebrospinal fluid barrier (BCSFB), which plays an important role as well. The barrier function is mainly a result of the functionality of the cerebral endothelial cells and choroidal epithelial cells in the choroid plexus. These cell types have restricted permeability due to the presence of tight junctions,3 and the concentration of a drug within the cerebrospinal fluid (CSF) primarily depends on how easy it can cross the BCSFB. Additionally, different transport mechanisms are available that control the exchange of compounds across the blood-brain barrier.4,5 The permeability itself depends on factors such as lipophilicity, molecular size, and plasma protein binding.6,7
Pharmacologic strategies to modify drugs to make them more lipophilic (eg, by replacing hydrophilic side groups with hydrophobic groups or by masking them with hydrophobic groups) have not been successful as these lipidized drugs distribute widely and become substrates for efflux mechanisms such as P-glycoprotein.8 Brain-to–blood transfer mechanisms are an important defense for the brain but a great impediment for drug entry. By restricting the entry into the CNS for a large number of drugs, P-glycoprotein remains a selective gatekeeper of the blood-brain barrier and thus a primary obstacle to drug delivery into the brain, contributes to the poor success rate of CNS drug candidates, and probably causes patient-to–patient variability in response to CNS pharmacotherapy.9
The blood-brain barrier and the BCSFB have a comparable functional organization with regard to the transport of molecules: they express various proteins in their membranes that either use carrier-mediated transcellular transport of solutes, maintaining optimal composition of the brain interstitial fluid, or use ATP-driven efflux of lipophilic molecules.10 Transport of solutes can be mediated by a superfamily of solute carriers (SLC), or ATP-binding cassette proteins directly couple efflux transport of molecules from a lipid bilayer.11
In order to ensure personalized psychopharmacotherapy, therapeutic drug monitoring, ie, the quantification of serum or plasma concentrations of medications for dose optimization, has proven a valuable tool.12 The clinical response to antidepressant drugs is known to depend on both plasma concentrations and concentrations at their target structures within the brain, and it is assumed that the concentration of a drug in CSF is an estimate of its blood-brain barrier permeability13 thereby reflecting the clinical response to an antidepressant treatment.14 Concentration values in CSF are used as surrogates for the unbound brain concentration of a drug in the interstitial fluid in the brain and therefore available for drug targets.15,16 However, the concentration of a drug in CSF more likely reflects its entry across the choroid plexus and the cerebrovascular route,8 but the clinical impact of CSF penetration remains unclear.
Venlafaxine extended release (XR) is a selective serotonin- and norepinephrine-reuptake inhibitor with antidepressant and anxiolytic properties. Venlafaxine XR is an extended-release capsule for oral administration that contains venlafaxine hydrochloride. Its molecular weight is 313.87 g/mol. Venlafaxine is metabolized by CYP isoenzymes, principally by CYP2D6 to O-desmethylvenlafaxine, its major active metabolite, but also by CYP2C19, CYP3A4, and CYP2C9. Likewise, venlafaxine is a relatively weak inhibitor of CYP2D6. The degree of binding of venlafaxine to human plasma is 27% ± 2% and 30% ± 12% for O-desmethylvenlafaxine.17
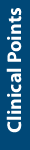
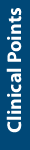
- During antidepressant treatment with venlafaxine, concentrations of the parent compound and its main metabolite O-desmethylvenlafaxine in cerebrospinal fluid (CSF) are relatively high compared to other psychotropic drugs, reaching 70%-90% of the concentrations obtained in plasma.
- Concentrations of venlafaxine and O-desmethylvenlafaxine in plasma rather than daily doses represent excellent indices for concentrations in CSF.
- Plasma concentrations of venlafaxine and O-desmethylvenlafaxine constitute biomarkers for CNS drug concentrations during antidepressant treatment.
The aim of the present study was to investigate the relationship between applied daily doses of venlafaxine XR and plasma as well as CSF concentrations of the parent compound venlafaxine and its active metabolite O-desmethylvenlafaxine in patients with depressive disorders under naturalistic conditions. Furthermore, the correlation between the measured plasma levels of both venlafaxine and O-desmethylvenlafaxine and CSF concentrations were calculated to account for the BCSFB penetration.
METHOD
Patients
This naturalistic observational study was carried out between November 2011 and August 2013 at the Department of Psychiatry, Psychotherapy, and Psychosomatics and the Department of Neurology at the University Hospital of RWTH Aachen University, Aachen, Germany. The local ethics committee approved the study protocol.
Sixteen patients of both sexes (10 women, 6 men), ranging in age from 35 to 81 years (mean age = 60.88 ± 16.20), diagnosed with a major depressive disorder, single episode or recurrent (ICD-10 criteria), that were on a stable therapy with venlafaxine XR with fixed daily doses for at least 7 days (daily doses between 75 and 225 mg, mean = 152.34 ± 67.73 mg/d), were included in the study. Patients underwent lumbar puncture during diagnostic workup to rule out an organic cause of the symptoms such as cognitive decline or to verify somatic symptoms such as sensory symptoms or deficits and not for the purpose of this study. All subjects underwent an extensive physical and laboratory check-up, including hematology, clinical chemistry, and electrocardiogram as part of the diagnostic routine program. Due to the observational character of the study, therapeutic drug monitoring was done in the remnants of CSF and plasma.
Only subjects with normal CSF status were included within the study, using the following criteria: no pleocytosis; no intrathecal IgG, IgM, or IgA synthesis according to the Reiber quotient charts18; no detection of oligoclonal bands; and no blood contamination at all.
Method
For application of the method to the analysis of plasma or serum, blood samples were taken from patients who were on a stable dose of venlafaxine XR for at least 7 days. Lumbar puncture was done to exclude conditions of acute infection, such as pleocytosis and elevated intrathecal albumin levels (elevated albumin quotient) as a sign of disturbances in BCSFB, and chronic infection, such as intrathecal synthesis of immunoglobulins (oligoclonal bands), as part of clinical routine without focusing on trough levels of the ingested drug. Blood was taken at the same time as lumbar puncture and was done primarily for the assessment of an intrathecal immunoglobulin synthesis to exclude a barrier dysfunction. Serum or plasma was prepared by centrifugation of blood samples at 5,000 rpm for 10 minutes and, if not assayed on the same day, stored at -32°C. Venlafaxine and O-desmethylvenlafaxine levels were determined by using high-performance liquid chromatography for simultaneous determination of venlafaxine and O-desmethylvenlafaxine as described previously.19,20 The lower limit of quantification was 10 ng/mL for both analytes, venlafaxine and O-desmethylvenlafaxine.
Statistical Analysis
To account for the range of doses of venlafaxine received by the patients, we first divided plasma levels of venlafaxine + O-desmethylvenlafaxine (active moiety [AM]) by the applied venlafaxine dose, resulting in a venlafaxine + O-desmethylvenlafaxine plasma level-by-dose ratio. Additionally, the penetration ratio into CSF for venlafaxine, O-desmethylvenlafaxine, and the AM were calculated. For this purpose, the levels of venlafaxine, O-desmethylvenlafaxine, and the AM in CSF were divided by their levels in plasma, reflecting the ratio of the particular compound that penetrated into CSF.
Correlations were computed to assess the relation between the level of drug concentration in plasma and CSF, as well as the relation of these concentrations with the daily dosage of venlafaxine. All variables of drug concentration were tested for normality of distribution using the Kolmogorov-Smirnov test. Pearson correlation was used in case of normally distributed data and Spearman correlation in case of non-normally distributed data.
All statistical analyses were conducted with SPSS (version 20, IBM, Armonk, New York).
RESULTS
The data on daily doses and plasma and CSF concentrations of venlafaxine and its active metabolite O-desmethylvenlafaxine are displayed in Table 1. Venlafaxine was applied in a mean daily dosage of 152.34 mg ± 67.73 mg (range, 75-225 mg). Since the dose was not normally distributed, Spearman correlation was used. With the exception of one patient (patient 14, AM: 98 ng/mL), all patients showed sufficient plasma levels within the recommended therapeutic reference range of 100-400 ng/mL12 or above. The relatively high ratios of venlafaxine > O-desmethylvenlafaxine (mean = 1.52, SD = 2.20; range, 0.20-8.74) might be explained by the time of sample collection. In most of the cases, analyses were done some hours after the intake of the medication and not around trough level time points. However, by analyzing patients 2, 6, 9, 10, and 11 (see Table 1) in the course of their treatment (detached from the study), more than 1 measured trough level of venlafaxine for patient 2 with a ratio below 1 suggests that she might be phenotypically an extensive metabolizer for cytochrome P450 (CYP) 2D6.19,21 Different trough levels for patients 6, 9, 10, and 11 with a ratio of venlafaxine/O-desmethylvenlafaxine above 1 suggest that these patients might phenotypically be intermediate metabolizers or less likely poor metabolizers.19,22 Due to the observational approach of the study, the patients were not genotyped for CYP 2D6. Plasma levels of venlafaxine ranged from 20.00 to 530.00 ng/mL (mean = 171.25 ng/mL, SD = 145.64 ng/mL) and O-desmethylvenlafaxine ranged from 19.00 to 503.00 ng/mL (mean = 197.38 ng/mL, SD = 145.36 ng/mL). The AM in plasma was on average 368.63 ng/mL (SD = 214.29 ng/mL; range, 98.00-675.00 ng/mL). A significant correlation of the daily venlafaxine dose and the venlafaxine plasma level could be observed (r = 0.659, P = .005). In addition, a trend was seen for the correlation of the concentration of the AM with the oral venlafaxine dose, but this effect failed to reach significance (plasma: r = 0.433, r2 = 0.187, P = .094; see Figure 1). Concentrations of O-desmethylvenlafaxine were not significantly correlated to the daily dose of venlafaxine. The mean level by dose ratio for the AM in plasma was 2.66 (SD = 1.78).
CSF levels of venlafaxine ranged from 11.00 to 507.00 ng/mL (mean = 133.69 ng/mL, SD 148.54 ng/mL), and O-desmethylvenlafaxine ranged from 26.00 to 380.00 ng/mL (mean = 155.88 ng/mL, SD = 105.08 ng/mL). The AM in CSF ranged from 58.00-711.00 ng/mL (mean = 289.56 ng/mL, SD = 178.07 ng/mL). The mean level by dose ratio for the AM in CSF was 2.06 (SD = 1.32).
The level of venlafaxine in CSF showed a significant correlation to the daily dose of venlafaxine (r = 0.562, r2 = 0.316, P = .023). However, no correlation of CSF levels of O-desmethylvenlafaxine with the daily dose of venlafaxine could be observed. A trend of a correlation of the AM in CSF with the dose of venlafaxine was detected (r = 0.480, r2 = 0.230, P = .060; see Figure 1).
Both the AM as well as the single parameters in plasma and CSF showed strong correlations (AM plasma and CSF: r = 0.835, r2 = 0.697, P < .001; venlafaxine plasma and CSF: r = 0.848, r2 = 0.719, P < .001; O-desmethylvenlafaxine plasma and CSF: r = 0.960, r2 = 0.922, P < .001; Pearson correlation). Nevertheless, the differences between the concentrations in plasma and CSF were significant for O-desmethylvenlafaxine (t15 = 3.106; P = .007) and the AM (t15 = 2.685; P = .017) with higher levels of concentration in plasma. For the concentration of venlafaxine, a similar trend could be observed, but this did not reach statistical significance (t15 = 1.855; P = .083).
The CSF/plasma ratio for venlafaxine varied from 0.23 to 1.93 (mean = 0.74, SD = 0.40); for O-desmethylvenlafaxine, it ranged from 0.48 to 1.56 (mean = 0.88, SD = 0.28), and for the AM, it varied from 0.40 to 1.87 (mean = 0.84, SD = 0.35; Figure 2).
DISCUSSION
Only some studies with small numbers of participants addressed the question as to what extent plasma concentrations of psychotropic drugs correlate with CSF levels. Knowledge is lacking concerning the impact of CSF drug levels on clinical outcome or on (cellular) homeostasis within CSF.23 For citalopram, CSF/plasma ratios were found to be 52% ± 9%; and for escitalopram, ratios were 48% ± 6%.24 For doxepin and its active metabolite, nordoxepin, significant correlations suggest a constant CSF permeability.25 Quetiapine and norquetiapine were found with a CSF/plasma ratio of only 3% ± 2% for quetiapine and 2% ± 2% for norquetiapine.26 CSF concentrations of olanzapine and desmethylolanzapine were 12% and 16%, respectively, of those in serum.27
Although some studies addressed the distribution pattern between plasma and CSF for different psychotropic drugs, data for venlafaxine and its metabolite O-desmethylvenlafaxine are missing. Therefore, this observational study is the first investigation of the distribution pattern of venlafaxine and its active metabolite O-desmethylvenlafaxine in plasma and CSF. Lumbar puncture and blood investigation were done for medical reasons unrelated to this study under stable daily doses of venlafaxine. Furthermore, the correlation between the measured plasma levels and CSF levels were calculated as an expression of the CSF penetration of venlafaxine and O-desmethylvenlafaxine.
We found a highly significant correlation between daily doses of venlafaxine and the concentration of venlafaxine in plasma and CSF. O-desmethylvenlafaxine did not show a significant correlation to the daily dose, and only a poor correlation between the AM (venlafaxine + O-desmethylvenlafaxine) and the daily dose of venlafaxine was found. Both compounds, venlafaxine + O-desmethylvenlafaxine, obviously make slightly different contributions to the antidepressant effect of venlafaxine in that higher plasma levels of O-desmethylvenlafaxine in extensive metabolizers are associated with a better clinical outcome.21 Hence, the observation of poor correlations between daily dosage, O-desmethylvenlafaxine, and the AM underscores that the measuring of plasma levels using therapeutic drug monitoring is more effective than (only) dose titration in order to achieve clinical efficacy. According to findings from positron emission tomography (PET) by Meyer et al,28 it can be assumed that all patients in our study had sufficiently high venlafaxine and O-desmethylvenlafaxine plasma levels to achieve antidepressant effects by occupying more than 80% of the SERT transporters.
We also found a strong and highly significant correlation between plasma and CSF concentrations of venlafaxine, O-desmethylvenlafaxine, and the AM in all of our patients that were in a stable psychopathological condition with a response or remission of a depressive episode under the ongoing antidepressant treatment. The CSF/plasma ratio for venlafaxine was found to be 0.74; for O-desmethylvenlafaxine, 0.88; and for the AM, 0.84. The significant correlation between and the high values for the ratios indicates a constant permeability for both compounds into CSF. These levels seem to be extraordinarily high, and comparable ratios for other psychotropic drugs have not been published so far.23,25,26,29,30 Our observation may be explained by the low plasma protein binding of venlafaxine and O-desmethylvenlafaxine.
Besides the fact that this good CSF/plasma correlation indicates that the BCSFB is highly permeable for venlafaxine and O-desmethylvenlafaxine, both compounds obviously do not undergo rapid elimination out of the CSF by (active) transport mechanisms. As none of the patients was a nonresponder in the sense of missing efficacy against depressive symptoms, it might be assumed that the high concentrations of the active compounds correlate with sufficient levels of both compounds at their target structures within the brain.
Since cell boundary structures such as the blood-brain barrier and the BCSFB are generally obstacles in the way of psychotropic drugs reaching their target structures, and only the free drug concentration is found in CSF, the plasma protein binding plays an important role. The plasma protein binding is low for venlafaxine (27%) and for O-desmethylvenlafaxine (30%). However, venlafaxine—like other psychotropic drugs, in particular antidepressant drugs—shows a variable and unpredictable therapeutic efficacy. The question arises whether drug concentrations in plasma can be considered as a valid surrogate marker of concentrations in brain/CSF following the hypothesis that the clinical response to antidepressant drugs correlates to their concentration in CSF.14,31
Our results are in-line with recent studies by Nikisch et al26 who compared plasma and CSF concentrations of quetiapine and showed that the CSF concentrations of the antipsychotic drug quetiapine and its metabolite norquetiapine correlate with those in plasma of schizophrenic patients. However, in case of quetiapine and its metabolite norquetiapine, the CSF/plasma ratio reached only about 3% and 2%, respectively.26 Nonetheless, the levels of both quetiapine and norquetiapine in serum and CSF correlated significantly with treatment response in terms of a decrease in the PANSS. In an earlier study, Nikisch et al had reported higher CSF/plasma ratios for citalopram24 although plasma protein binding of citalopram is high (50%-80%) in comparison to venlafaxine and O-desmethylvenlafaxine.32 Schomburg et al25 reported CSF concentrations for doxepin and nordoxepin of 11% of the plasma concentration in accordance with the assumption that the CSF concentration reflects the unbound fraction of the drug.
These results, taken together, indicate that CSF sampling is of high clinical value. It is the only generally applicable method to obtain information on free drug concentrations in individual human brains. Since the ultimate interest is in the free drug concentration at the CNS target site, the question is what CSF concentrations may tell us in that respect.33 To interpret CSF drug levels as an indicator of adequate transport of a drug into the CNS, it is necessary to investigate the regulation of drug distribution between the brain and CSF.33 For obvious reasons, available data on relationships between drug concentrations in CSF and brain extracellular fluid (ECF) in humans are extremely rare. They were obtained in only 1 study intraoperatively from patients with intractable epilepsy.34 In that study, carbamazepine, 10-hydroxy-carbazepine (10-OH-CZ, the active metabolite of oxcarbazepine), lamotrigine, and levetiracetam were investigated. It was found that CSF concentrations were significantly higher than corresponding brain ECF concentrations. This ratio was ~2.5 for carbamazepine, ~3.5 for both 10-OH-CZ and levetiracetam, and ~4.0 for lamotrigine. In order to be able to more accurately predict human brain ECF concentrations, our understanding of CNS complexity in terms of intrabrain pharmacokinetic relationships, brain distribution, and the influence of CNS disorders needs to be improved.
Finally, it is important to notice that venlafaxine is chiral. It is known that the different enantiomers display different pharmacodynamic profiles in inhibiting presynaptic reuptake at different target structures. R(-)-venlafaxine inhibits norepinephrine and serotonin reuptake; S(+)-venlafaxine primarily inhibits serotonin reuptake.35 A difference in the distribution of the enantiomers according to CYP2D6 metabolizer status36 might be of importance when interpreting the results; however, a chiral analysis has not been performed in our study. An enantioselective analysis of venlafaxine and its metabolites could, in this regard, assist in the interpretation of the distribution pattern between plasma and CSF.
CONCLUSIONS
In the clinical setting, drug concentrations in CSF are sometimes used as a surrogate for drug concentrations at the target site within the brain. However, the brain consists of multiple compartments, and many factors are involved in the transport of drugs from plasma into the brain and the distribution within the brain. In particular, active transport processes at the level of the blood-brain barrier and BCSFB, such as those mediated by P-glycoprotein, lead to complex relationships between concentrations in plasma, ventricular and lumbar CSF, and other brain compartments. In our study, we found very high levels of venlafaxine and O-desmethylvenlafaxine suggesting a high permeability for both compounds into CSF. However, the question arises whether the clinical outcome of antidepressant treatment is necessarily attached to high CSF levels of antidepressant drugs. Only the correlation between the clinical outcome and CSF levels of psychotropic drugs could provide information about the value of measured CSF levels. Without this clinically important information, CSF concentrations of psychotropic drugs may be difficult to interpret and may have limited value. As a next step, further information about CSF concentrations of antidepressant drugs and predictors of antidepressant treatment, such as proinflammatory cytokines, could be helpful to understand the interplay on a molecular basis.
Limitations
High concentrations of venlafaxine and O-desmethylvenlafaxine in CSF indicate good availability of the drug in the brain. The findings, however, do not allow conclusions to be drawn on concentrations that can be expected at the target structure within the brain.
Drug names: carbamazepine (Carbatrol, Equetro, and others), citalopram (Celexa and others), doxepin (Silenor, and others), escitalopram (Lexapro and others), lamotrigine (Lamictal and others), levetiracetam (Keppra and others), olanzapine (Zyprexa), oxcarbazepine (Trileptal and others), quetiapine (Seroquel), venlafaxine (Effexor and others).
Author affiliations: Department of Psychiatry, Psychotherapy, and Psychosomatics, RWTH Aachen University, and JARA-Jülich Aachen Research Alliance-Translational Brain Medicine, Jülich/Aachen (Drs Paulzen, Groppe, Veselinovic, and Gründer); Department of Neurology, RWTH Aachen University, Aachen (Dr Tauber); and Neurochemical Laboratory, Department of Psychiatry and Psychotherapy, University Medical Center of Mainz, Mainz (Dr Hiemke), Germany.
Author contributions: Participated in research design: Drs Paulzen, Hiemke, and Gründer; conducted experiments: Drs Paulzen, Tauber, and Veselinovic; contributed analytic tools: Dr Hiemke; performed data analysis: Drs Paulzen and Groppe; and wrote or contributed to the writing of the manuscript: all authors.
Potential conflicts of interest: Dr. Gründer has served as a consultant for Bristol-Myers Squibb, Cheplapharm, Eli Lilly, Forest, Lundbeck, Otsuka, Roche, Servier, and Takeda; has served on the speakers’ bureau of Bristol-Myers Squibb, Eli Lilly, Gedeon Richter, Otsuka, Roche, and Servier; has received grant support from Alkermes, Bristol-Myers Squibb, Eli Lilly, and Roche; and is cofounder of Pharma-Image-Molecular Imaging Technologies GmbH. Dr. Hiemke has served as a consultant for Servier and Janssen-Cilag; has served on the speakers’ bureau of Bristol-Myers Squibb, Eli Lilly, Pfizer, and Servier; and is managing director of the psiac GmbH, Mainz, which provides an Internet-based drug interaction program for psychoactive drugs. Drs Paulzen, Groppe, Tauber, and Veselinovic have no financial interests to declare.
Funding/support: None reported.
Acknowledgments: The authors thank Anette Rieger-Gies, Sandra Heller, and Gaby Stroba from the Neurochemical Laboratory at the University Medical Center at Mainz for determination of venlafaxine and O-desmethylvenlafaxine for this investigation. The acknowledged individuals report no conflict of interest.
REFERENCES
1. Löscher W, Potschka H. Drug resistance in brain diseases and the role of drug efflux transporters. Nat Rev Neurosci. 2005;6(8):591-602. PubMed doi:10.1038/nrn1728
2. Sakaeda T, Nakamura T, Okumura K. Pharmacogenetics of drug transporters and its impact on the pharmacotherapy. Curr Top Med Chem. 2004;4(13):1385-1398. PubMed doi:10.2174/1568026043387692
3. de Lange EC. Potential role of ABC transporters as a detoxification system at the blood-CSF barrier. Adv Drug Deliv Rev. 2004;56(12):1793-1809. PubMed doi:10.1016/j.addr.2004.07.009
4. Begley DJ, Bradbury MWB, Kreuter J. The Blood-Brain Barrier and Drug Delivery to the CNS. New York, NY: Dekker; 2000.
5. Abbott NJ. Evidence for bulk flow of brain interstitial fluid: significance for physiology and pathology. Neurochem Int. 2004;45(4):545-552. PubMed doi:10.1016/j.neuint.2003.11.006
6. de Boer AG, Gaillard PJ. Drug targeting to the brain. Annu Rev Pharmacol Toxicol. 2007;47(1):323-355. PubMed doi:10.1146/annurev.pharmtox.47.120505.105237
7. Yasuda K, Cline C, Vogel P, et al. Drug transporters on arachnoid barrier cells contribute to the blood-cerebrospinal fluid barrier. Drug Metab Dispos. 2013;41(4):923-931. PubMed doi:10.1124/dmd.112.050344
8. Saunders NR, Ek CJ, Habgood MD, et al. Barriers in the brain: a renaissance? Trends Neurosci. 2008;31(6):279-286. PubMed doi:10.1016/j.tins.2008.03.003
9. Miller DS, Bauer B, Hartz AMS. Modulation of P-glycoprotein at the blood-brain barrier: opportunities to improve central nervous system pharmacotherapy. Pharmacol Rev. 2008;60(2):196-209. PubMed doi:10.1124/pr.107.07109
10. Jones HC, Terasaki T. Fluids and Barriers of the CNS: a new journal encompassing Cerebrospinal Fluid Research. Fluids Barriers CNS. 2011;8(1):1. PubMed
11. Dean M, Hamon Y, Chimini G. The human ATP-binding cassette (ABC) transporter superfamily. J Lipid Res. 2001;42(7):1007-1017. PubMed
12. Hiemke C, Baumann P, Bergemann N, et al. AGNP consensus guidelines for therapeutic drug monitoring in psychiatry: update 2011. Pharmacopsychiatry. 2011;44(6):195-235. doi:10.1055/s-0031-1286287
13. Morrey JD, Olsen AL, Siddharthan V, et al. Increased blood-brain barrier permeability is not a primary determinant for lethality of West Nile virus infection in rodents. J Gen Virol. 2008;89(pt 2):467-473. PubMed doi:10.1099/vir.0.83345-0
14. Nordin C, Bertilsson L. Active hydroxymetabolites of antidepressants: emphasis on E-10-hydroxy-nortriptyline. Clin Pharmacokinet. 1995;28(1):26-40. PubMed doi:10.2165/00003088-199528010-00004
15. Liu X, Van Natta K, Yeo H, et al. Unbound drug concentration in brain homogenate and cerebral spinal fluid at steady state as a surrogate for unbound concentration in brain interstitial fluid. Drug Metab Dispos. 2009;37(4):787-793. PubMed doi:10.1124/dmd.108.024125
16. Kodaira H, Kusuhara H, Fujita T, et al. Quantitative evaluation of the impact of active efflux by p-glycoprotein and breast cancer resistance protein at the blood-brain barrier on the predictability of the unbound concentrations of drugs in the brain using cerebrospinal fluid concentration as a surrogate. J Pharmacol Exp Ther. 2011;339(3):935-944. PubMed doi:10.1124/jpet.111.180398
17. Effexor XR (venlafaxine HCl) Extended-Release [package insert]. Philadelphia, PA: Pfizer; 2012 http://www.accessdata.fda.gov/drugsatfda_docs/label/2004/20151slr028,030,032,20699slr
041,048,052_effexor_lbl.pdf. Accessed March 31, 2014.
18. Reiber H, Felgenhauer K. Protein transfer at the blood cerebrospinal fluid barrier and the quantitation of the humoral immune response within the central nervous system. Clin Chim Acta. 1987;163(3):319-328. PubMed doi:10.1016/0009-8981(87)90250-6
19. Shams ME, Arneth B, Hiemke C, et al. CYP2D6 polymorphism and clinical effect of the antidepressant venlafaxine. J Clin Pharm Ther. 2006;31(5):493-502. PubMed doi:10.1111/j.1365-2710.2006.00763.x
20. Hicks DR, Wolaniuk D, Russell A, et al. A high-performance liquid chromatographic method for the simultaneous determination of venlafaxine and O-desmethylvenlafaxine in biological fluids. Ther Drug Monit. 1994;16(1):100-107. PubMed doi:10.1097/00007691-199402000-00016
21. Lobello KW, Preskorn SH, Guico-Pabia CJ, et al. Cytochrome P450 2D6 phenotype predicts antidepressant efficacy of venlafaxine: a secondary analysis of 4 studies in major depressive disorder. J Clin Psychiatry. 2010;71(11):1482-1487. PubMed doi:10.4088/JCP.08m04773blu
22. Hicks JK, Swen JJ, Thorn CF, et al; Clinical Pharmacogenetics Implementation Consortium. Clinical Pharmacogenetics Implementation Consortium guideline for CYP2D6 and CYP2C19 genotypes and dosing of tricyclic antidepressants. Clin Pharmacol Ther. 2013;93(5):402-408. PubMed doi:10.1038/clpt.2013.2
23. Baumann P, Jonzier-Perey M, Paus E, et al. Mirtazapine enantiomers in blood and cerebrospinal fluid. Neuropsychobiology. 2006;54(3):179-181. PubMed doi:10.1159/000098654
24. Nikisch G, Mathé AA, Czernik A, et al. Stereoselective metabolism of citalopram in plasma and cerebrospinal fluid of depressive patients: relationship with 5-HIAA in CSF and clinical response. J Clin Psychopharmacol. 2004;24(3):283-290. PubMed doi:10.1097/01.jcp.0000125680.89843.a6
25. Schomburg R, Remane D, Fassbender K, et al. Doxepin concentrations in plasma and cerebrospinal fluid. J Neural Transm. 2011;118(4):641-645. PubMed doi:10.1007/s00702-011-0613-x
26. Nikisch G, Baumann P, Wiedemann G, et al. Quetiapine and norquetiapine in plasma and cerebrospinal fluid of schizophrenic patients treated with quetiapine: correlations to clinical outcome and HVA, 5-HIAA, and MHPG in CSF. J Clin Psychopharmacol. 2010;30(5):496-503. PubMed doi:10.1097/JCP.0b013e3181f2288e
27. Skogh E, Sjödin I, Josefsson M, et al. High correlation between serum and cerebrospinal fluid olanzapine concentrations in patients with schizophrenia or schizoaffective disorder medicating with oral olanzapine as the only antipsychotic drug. J Clin Psychopharmacol. 2011;31(1):4-9. PubMed doi:10.1097/JCP.0b013e318204d9e2
28. Meyer JH, Wilson AA, Sagrati S, et al. Serotonin transporter occupancy of five selective serotonin reuptake inhibitors at different doses: an [11C]DASB positron emission tomography study. Am J Psychiatry. 2004;161(5):826-835. PubMed doi:10.1176/appi.ajp.161.5.826
29. Nikisch G, Mathé AA, Czernik A, et al. Long-term citalopram administration reduces responsiveness of HPA axis in patients with major depression: relationship with S-citalopram concentrations in plasma and cerebrospinal fluid (CSF) and clinical response. Psychopharmacology (Berl). 2005;181(4):751-760. PubMed doi:10.1007/s00213-005-0034-3
30. Little JT, Ketter TA, Mathé AA, et al. Venlafaxine but not bupropion decreases cerebrospinal fluid 5-hydroxyindoleacetic acid in unipolar depression. Biol Psychiatry. 1999;45(3):285-289. PubMed doi:10.1016/S0006-3223(98)00078-X
31. Sathananthan GL, Gershon S, Almeida M, et al. Correlation between plasma and cerebrospinal levels of imipramine. Arch Gen Psychiatry. 1976;33(9):1109-1110. PubMed doi:10.1001/archpsyc.1976.01770090099009
32. Hiemke C, Hפrtter S. Pharmacokinetics of selective serotonin reuptake inhibitors. Pharmacol Ther. 2000;85(1):11-28. PubMed doi:10.1016/S0163-7258(99)00048-0
33. de Lange EC. Utility of CSF in translational neuroscience. J Pharmacokinet Pharmacodyn. 2013;40(3):315-326. PubMed doi:10.1007/s10928-013-9301-9
34. Rambeck B, Jürgens UH, May TW, et al. Comparison of brain extracellular fluid, brain tissue, cerebrospinal fluid, and serum concentrations of antiepileptic drugs measured intraoperatively in patients with intractable epilepsy. Epilepsia. 2006;47(4):681-694. PubMed doi:10.1111/j.1528-1167.2006.00504.x
35. Holliday SM, Benfield P. Venlafaxine: a review of its pharmacology and therapeutic potential in depression. Drugs. 1995;49(2):280-294. PubMed doi:10.2165/00003495-199549020-00010
36. Eap CB, Lessard E, Baumann P, et al. Role of CYP2D6 in the stereoselective disposition of venlafaxine in humans. Pharmacogenetics. 2003;13(1):39-47. PubMed doi:10.1097/00008571-200301000-00006